Helion’s fusion system is (basically) an RLC circuit
Manav Singh, Senior Manager of Electrical Engineering
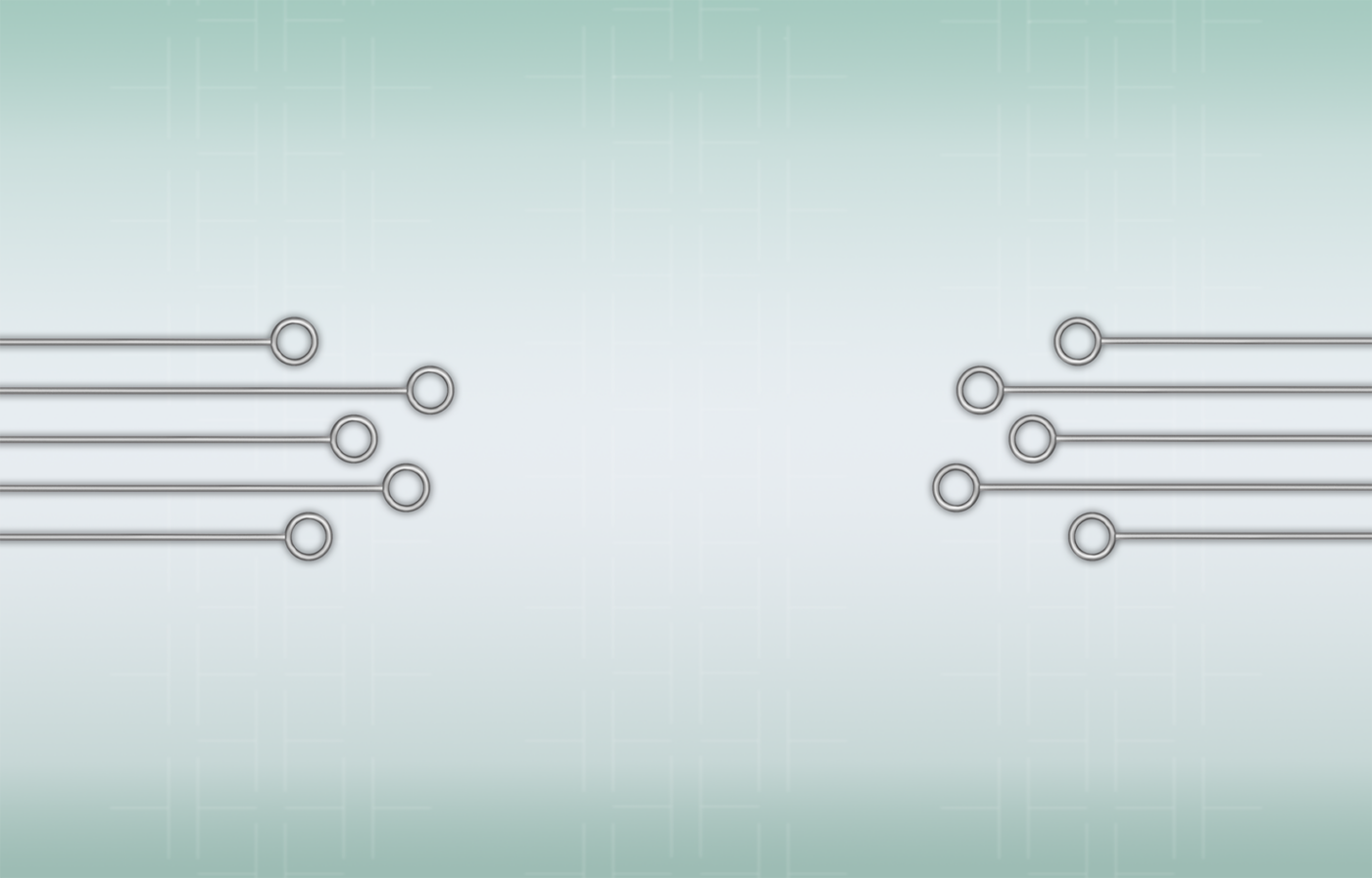
If you’ve touched a breadboard or opened an EE textbook, you’ve met the RLC circuit. It’s a simple electrical loop containing three elements: a resistor (R), an inductor (L), and a capacitor (C).
Adding a switch provides a mechanism for controlling the flow of energy in the loop into familiar waveforms – a sine wave for current and a cosine wave for capacitor voltage on the capacitor (technically, a damped sine and cosine). In this RLC circuit the capacitor discharges energy through the switch into the inductor, where energy builds up before dissipating back to the capacitor. This cycle repeats until the resistor dissipates all the energy into heat, or the circuit is made to stop conducting current.
Helion approaches fusion technology by taking that simple electrical engineering concept – oscillating energy through inductance and capacitance – and scaling it way up.
Basically, we’re building large RLC circuits.
Imagine several huge copies of this circuit. With capacitors larger than most side tables and inductors so large they can only be moved with overhead cranes. All within a space as big as a small warehouse. In Polaris’ case, a 30,000 sq. ft. facility made from slabs of concrete.
Our capacitor bank – C – is made up of thousands of high voltage pulsed capacitors, capable of storing over 50 megajoules of energy in total. Our inductors – L – are a cylindrical skeleton of electromagnetic coils that make up Polaris’ mechanical build. Each of the inductors is connected to hundreds of capacitors, making it effectively lots of separate, but interconnected, RLC circuits. We charge the capacitor bank to tens of thousands of volts, then discharge the energy into the electromagnetic coils through a massive network of switches and coaxial cables. The switches and cables (technically, the coils themselves as well) contribute to the majority of the losses – R.
Here’s where an extra element is added. As energy moves from the electric fields in the capacitors to the magnetic fields in the inductors, we use those magnetic fields to form, accelerate, and compress our plasmas to fusion conditions. As the plasma and the circuit interact, fusion occurs and induces a current back into the electromagnetic coils, which travels through the system’s cables and switches, and back into the capacitor bank.
Our circuits provide a more efficient path to generating electricity from fusion.
Traditional fusion machines tend to rely on steady state operation and confinement with complex magnetic geometries with immense cooling systems. Most aim to heat plasma until it fuses and then use the resulting heat to boil water and spin a turbine – essentially making a complicated steam engine.
Helion’s approach bypasses that. The system is inherently electrical. There is no need to convert heat into motion and back into electricity. Instead, the machine pulses, fuses, and recharges its own energy bank, while producing excess electricity that can be sold.
In turn, we inherently surpass efficiency limits set by steam cycles. This comes with fewer systems and requires less fusion, meaning the entire architecture can be smaller, simpler, and more modular.
And this really is important – especially in the context in which Helion is building. At the end of the day, our goal is not to design the most perfect circuit or achieve the highest fusion output. Our goal is to generate clean, reliable, and affordable electricity. Our team believes that direct electricity recapture from fusion is the best shot at solving that challenge. It minimizes the number of systems that need to be perfect and allows multiple avenues to increase output.
That does not mean it’s easy.
This plasma-to-circuit coupling introduces engineering challenges rarely encountered in conventional power systems. The plasma doesn’t just consume energy – it induces current back into the electromagnetic coils, turning the system into a bidirectional, tightly coupled electrical loop. Capturing that return energy efficiently requires precise control over timing, impedance, and switching behavior.
Adding plasma to the mix makes it more challenging (but also, more fun and interesting!) – plasmas are highly dynamic and inherently unstable. The circuit must tolerate variability in pulse shape and energy output, while protecting components from voltage spikes and current transients. Managing these dynamics pushes the limits of high-speed switching, real-time sensing, and integrated electromechanical design – all in service of maximizing electricity recapture.
We’re tackling hard engineering challenges with simple ideas.
I fully believe that for us to bring fusion electricity to the grid, we must aim for applying simple systems to hard problems. In some ways that’s what I find so compelling about Helion: We’re applying a simple electrical architecture – the RLC circuit – to one of the most complex challenges humanity has ever tried to solve, and we’re finding that it can work.
Sometimes the path to the future isn’t through more complexity – it’s through the right kind of simplicity.
As we continue to build the world’s best fusion machines, our focus will be on innovating to keep our systems as simple as possible without sacrificing performance. And as I’ve learned over time, it takes the best electrical engineers to do that.
If you’ve read this far and you’re an electrical engineer that loves first principles thinking and making a huge impact on the course of humanity– you should come build the future of fusion energy with us.